Ralf BECKER, Stefan HERLE, Rainer LEHFELDT, Peter
FRÖHLE, Jürgen JENSEN, Till QUADFLIEG, Holger SCHÜTTRUMPF and Jörg
BLANKENBACH, Germany
Early warning systems for floods and storm surges currently are based
exclusively on water level fore-casts. Other loads such as wind, waves,
currents or heavy rainfalls as well as the resistance of the flood
protection structure itself (e. g. dikes, flood protection walls) are
not considered. If they occur simultaneously, the flood protection
structures may fail before the design load is reached. Therefore, it is
absolutely necessary to develop a sensor- and risk based early warning
system which includes all relevant processes and cascading effects,
allows just in time warnings and provides reliable and robust real-time
data. A geoportal visualizing all collected data and information should
grant decision-makers access.
Our integrated approach involves the implementation of a Sensor and
Spatial Data Infrastructure (SSDI) enabling the concept of open access
to all relevant data, models and simulations. For the real-time data
collection at spatially distributed locations on-site, geotextiles are
installed in the dike structure and mounted to sensor nodes for
monitoring of deformations inside the dike. Furthermore, the nodes are
equipped with environmental sensors capturing additional required data
(e.g. soil temperature, humidity).
By using wireless communication protocols, a highly scalable and
flexible wireless geo sensor network is built up enabling large scale
monitoring of sea dikes. For maximizing the information effectiveness
and reducing the own efforts, additional data from existing
spatial-temporal data repositories (discoverable and accessible by web
services) can be coupled with the sensor data. This fusion and
integration of all relevant information facilitates a holistic analysis
for early warning. The use of (geo) standards ensures integration of
such heterogeneous data sources and interoperability. Current missing
(geospatial) standards for mandatory new functionalities will be
specified and developed. This gateway to the early warning system
enables access to the real-time sensor, other existing data resources as
well as to methods and results of hydro engineering simulation tools.
Thus, the geoportal allows user access to all information via Internet
with stationary or mobile clients at any time and any place.
1. INTRODUCTION
The German Federal Ministry of Education and Research (BMBF) and the
German Research Foundation (DFG) have identified the necessity to foster
methods for early detection of natural risks, especially intensified by
cascading effects and using innovative sensor and information
technologies.
One of the main hazards is the failure of coastal dikes triggered by
water and wind of the North Sea. Seadikes and estuarine dikes represent
the main coastal protection structures in Germany and protect low lying
areas in the northern federal states (e.g. Schleswig-Holstein). More
than 2,400,000 people and an area of more than 12,000 km² are protected
by more than 1,200 km of sea dikes and estuarine dikes in Germany
(Schüttrumpf, 2008). Although the affected landmass is not comparable to
flooded landmass in other parts of the world, the density of population
and economic value in Germany gives protection a high importance. For
example, in the city of Hamburg the protected value by estuarine dikes
is more than 10 Billions of Euro, in Schleswig-Holstein more than 47
Billions of Euro (Schüttrumpf, 2008). Taking these numbers into account,
it is obvious that the safety of these structures has a tremendous
relevance for all activities in coastal areas.
Nowadays, early warning systems are only based on water level
observations and predictions. It is well understood, that wind, waves,
currents and the resistance of sea dikes towards wind and waves play
also a pivotal role in dike safety. Therefore, it is required to
establish an early warning system taking all parts of the chain of
events within a risk based approach into account by combining different
sensor and third party data, prediction models, marine data
infrastructures and smart technologies. It should operate in real time,
allow access to all data and results at any time and any place and has
to consider international standards for data definition and exchange.
The presented project called “EarlyDike” focuses primarily on the
development of techniques and modeling tools which are required for an
innovative and intelligent risk- and sensor-based early warning-system
for coastal and estuarine dikes. Using these developments the German
authorities can fulfill their responsibility for up-to-date coastal
protection.
2. EarlyDike - A risk- and sensor-based
early warning-system
EarlyDike aims at supporting authorities in making smart just-in-time
decision based on relevant and processed information. Such an
intelligent risk- and sensor-based early warning-system needs several
different input data, indicators and simulators to decide about the risk
of a (dike) failure, its probability and its consequences of inundation
areas and the necessity of evacuation of population and protection of
economic values. The project is therefore divided up in 5 packages (Fig.
1):
- Storm Surge Monitor and Simulator
- Wave Monitor and Simulator
- Dike Monitor and Simulator
- Flood Simulator
- Geoportal, Sensor and Spatial Data
Infrastructure
The project requires different key competences and knowledge which leads
to an interdisciplinary team of research institutes working on the
different packages.
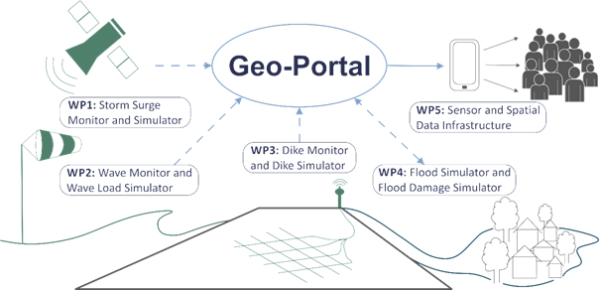
Fig. 1: Packages of EarlyDike-project
2.1 Storm Surge Monitor and Simulator
The “Storm Surge Monitor and Simulator” package covers the development
of a statistical storm surge model by using observation and model data
as a basis for a storm surge simulation. Mainly responsible for this
package is the Research Institute for Water and Environment at
University of Siegen (fwu) (Fig. 2). The work constitutes of water level
forecasts (up to three days ahead) for the entire German North Sea
coastline with a high temporal (at least 15-minute values) and, more
importantly, a high spatial resolution, i.e. continuously every few
kilometers along the coastal defense line will be provided. Optimized
tidal predictions for the shallow German Bight, point wise water level
forecasts of the Federal Maritime and Hydrographic Agency (BSH), and
wind forecasts from the German Weather Service (DWD) taken into account,
water level forecasts will be available for the entire German North Sea
coastline.
2.2 Wave Monitor and Simulator
Based on observations of e.g. wind or water level and the influence and
behavior of waves with a statistical wave model, a wave simulator is
developed. High accuracy wave impact nowcasts and forecasts as
operational systems with a temporal resolution of Δt = 1h and depending
on the local geomorphological formation with a spatial resolution of up
to a few 100m will be provided. The Institute of River and Coastal
Engineering at Hamburg’s University of Technology (TUHH WB) is
responsible leading partner of this package.
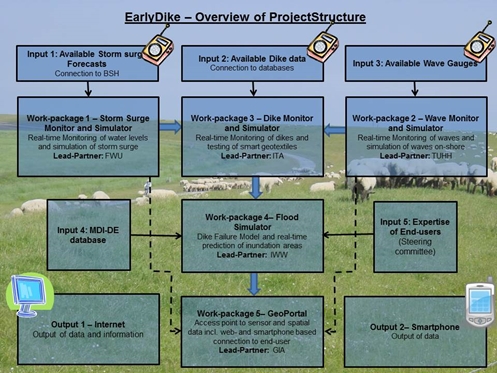
Fig. 2: EarlyDike: Organisation structure and work program
2.3 Dike Monitor and Simulator
In the third package the dike construction itself will be
real-time-monitored by sensors for soil temperature, soil moisture and
by newly developed smart “geotextiles”. Taking into account the
influences of soil temperature and soil moisture, the geotextile will be
able to identify stress and deformation (strain). Both, strain and
moisture can be measured by the change in electrical resistance of
special sensor yarns. The specification of the required configuration
and spatial resolution in the measurement points is integral part of the
research. This package is led by the Institut fuer Textiltechnik (ITA)
and the Institute of Hydraulic Engineering and Water Resources
Management (IWW) at the RWTH Aachen University.
2.4 Flood Simulator
The “Flood Simulator” is implemented by IWW. It computes predictions of
inundation areas in the case of an expected dike failure on the basis of
the simulators of the prior packages. The resolution of the computation
of the flood simulator solely depends on the geospatial data input.
2.5 GeoPortal, Sensor and Spatial Data Infrastructure
(SSDI)
An early warning system for dike failures and resulting flooding events,
as described in the subsections before, requires permanent monitoring of
changes of the physical parameters (waves, water levels, dike) and
forecast of changes and impacts. The working package, “GeoPortal, Sensor
and Spatial Data Infrastructure“ deals with the integration and fusion
of the geo sensor networks, simulations and other spatial data into a
single service-oriented architecture (SOA). In addition, dissemination
channels like a web portal will be realized. The Geodetic Institute and
Chair for Computing in Civil Engineering & Geo Information Systems at
the RWTH Aachen University (gia) and the Federal Waterways Engineering
and Research Institute (BSW) are the responsible partners for this
package. The GeoPortal is the interconnecting part for data input,
exchange and output. This paper focuses mainly on the integration of
data and results of the previously briefly described packages and the
setup of a web-based geoportal.
3. ASPECTS OF DATA INPUT, DATA EXCHANGE
AND DATA OUTPUT
The EarlyDike geoportal provides access to the input data for the
different simulators and models captured by in-situ sensors or received
from third parties. Further, a user interface is to be implemented
enabling the integration of the simulators and exploration of the
corresponding results.
Several institutions like the German Meteorological Service (DWD) or the
Federal Maritime and Hydrographic Agency of Germany (BSH) already offer
data which can be utilized to run our simulations. These datasets can be
retrieved on demand via data carriers or FTP server. Although there
exists already some standardized services, the main procedure of
requesting this data still involves high manual efforts which also lead
to issues in reliability and timeliness of data. A more sophisticated
approach implies using web services to reduce manual efforts and enhance
data quality. Therefore, the concept of Spatial Data Infrastructures
(SDI) is to be applied.
3.1 Spatial Data Infrastructures
Data networks for spatial information (SDI) are currently built up
worldwide improving the availability and the exchange of spatial related
data (Groot 2003, Bocher & Neteler 2012, Harvey et al. 2012). Generally,
a SDI provides user access to many voluminous geospatial datasets via a
consistent infrastructure (Schleyer et al. 2014). SDIs are currently
established in different levels by governments in the European Union
(EU) member states (Craglia & Annoni 2006, Thomas 2013) and for
different themes. The Germany-wide SDI „Geodateninfrastruktur
Deutschland” (GDI-DE) is a network of the state of Germany, its federal
members and local authorities for spatial data access. It is integrated
into the EU INSPIRE infrastructure. A current state report of GDI-DE is
published by the “Lenkungsgremium GDI-DE” (BKG 2013). Regarding waters
and coastal protection, the publicly funded marine spatial data
infrastructure Germany (MDI-DE) (Lehfeldt & Melles 2011, Rüh & Bill
2012) (fusing former NOKIS and GDI-BSH) integrates data resources for
coastal engineering, marine environment, and maritime conservation from
different German federal states and national agencies. Web portals are
often deployed to discover, view, access, and query geo information via
the Internet (Bernard et al. 2005). These so-called geoportals serve as
central access points to spatial data infrastructures, e.g. the
GeoPortal NRW or the portals of the Metropolregions Hamburg and Berlin.
Other existing geoportals provide access to georeferenced information of
different themes like energy, land use, etc. For the EarlyDike specific
data and simulation results, an own SDI will be set up and a geoportal
will be created to explore this data.
3.2 In-situ data - Wireless Sensor technology
Since the provided datasets by external SDIs are not sufficient as input
data for dike simulations, the seadike itself has to be enriched by
in-situ sensors capturing crucial observation values for monitoring and
simulating the inner state of the dike. The sensor data should be
transmitted in real-time to the database and the simulators.
Additionally, the monitoring stations should be spatially spread over
the whole dike to assure an area-covering monitoring. Since the
utilization of innovative geo textiles is a new concept in dike
monitoring, defining useful specifications for e.g. accuracy, timelines
and spatial resolution is part of the research. These requirements lead
to an architecture called Wireless Geo Sensor Network (WGSN). (Wireless)
sensor technology has rapidly developed in the past years and became
interesting for more and more disciplines. Current sensor nodes can be
equipped with multiple sensors for capturing environmental parameters
(e.g. air temperature, air pollution, water quality, etc.). Due to the
ongoing improvements, geo sensor networks are increasingly utilized to
monitor Earth’s phenomena. Examples are disaster management,
environmental monitoring, public security or urban flooding (Iyengar &
Brooks 2012, Akyildiz & Vuran 2010, Pengel et al. 2013). At each
measuring site the needed parameters (see 2.3)have to be collected. All
different kinds of sensors at one location are aggregated and mounted at
a single sensor node in our network.
3.3 Standardization
Using data of different sources, standards for data formats and exchange
are essential. Standardization initiatives aim at standardizing data
structures, data exchange and also sensor definition, description and
observation that enables data capturing and joining from different
sources.
In Europe the INSPIRE directive (INSPIRE 2007) aims to “establish an
infrastructure for spatial information in Europe to support Community
environmental policies, and policies or activities which may have an
impact on the environment” and is a legislative act for all EU member
states. Data structures defined by the INSPIRE directive and its
subsequent specification documents are related to the Open Geospatial
Consortium (OGC)-standards.
The OGC is a worldwide consortium of companies, government agencies and
universities participating in a consensus process to develop public
available interface standards. The standards empower technology
developers to make complex spatial information and services accessible
and useful with all kinds of applications[1]. In
particular the OGC web service interface standards (e.g. Web Map
Services (WMS), Web Feature Services (WFS)) (Benedict 2005) and the OGC
data encoding standards (e.g. GML, O&M, WaterML) are important for the
project’s objectives of data exchange and data fusion. For improving the
integration capacity of geo sensor resources in applications and
ensuring interoperability, the OGC originated the Sensor Web Enablement
Initiative (SWE) (Grothe & Kooijman 2008). SWE is occasionally used in
recent projects, such as OSIRIS utilized for different use cases (e.g.
air pollution monitoring) (Jirka et al. 2009), SLEWS for landslide early
warning (Walter & Nash 2009) or FluGGS for river basin management (Spies
& Heier 2010).
4. PROPOSED ARCHITECTURE
The package “Geoportal, Sensor and Spatial Data Infrastructure“ of the
EarlyDike project aims at the development of a Sensor and Spatial Data
Infrastructure (SSDI) for early warning (Fig. 3). It combines existing
spatial-temporal data captured by means of a WGSN. The data will be used
in different monitoring and simulation tools and will be provided
together with the simulation results to the end-user by a geoportal.
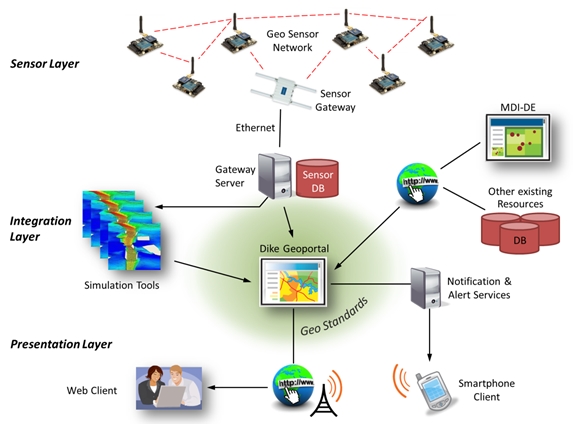
Fig. 3: Sensor and Spatial Data Infrastructure for dike monitoring
The fusion of multiple, heterogeneous data resources in an early warning
system is a crucial aspect for a holistic monitoring approach. Thus,
physical interfaces to the sensor data, soft-ware interfaces to the
external data resources as well as to the monitoring and simulation
tools have to be developed and implemented. Following the principal of
SOA for distributed software systems, web services are deployed. In
order to ensure interoperability, thereby international standards from
geo information science defined by INSPIRE of the European Union and the
OGC are adapted. Those standards will be – if necessary - extended to
hydro engineering simulation tasks.
As central access point to all information a dike geoportal is
implemented and deployed. It also enables access from smartphone devices
and introduces notification and alert services. Alert messages are
created automatically as result of the automatic real-time analysis of
the collected sensor data and the connected hydro engineering processing
tools. Alerts may not only be given for the present but also as a
forecast for future events.
Summarizing, the geoportal has to cover the following four aspects:
- Capturing and processing of in-situ data
of the sensor nodes
- Integration of external data sources
- Integration of tools for monitoring and
simulation
- Providing and visualization of the data
(row data, simulation results etc.)
4.1 Inventory of existing information and definition
of the requirements
The definitions of the data types needed and the specification of the
simulators’ requirements in regard to spatial and temporal resolution,
quality and accuracy are formulated in a previous step. Existing data
providers and SDIs are browsed for data supply and data accordance to
the predefined requirements.
There are plenty external data suppliers like the German authorities for
spatial base data of landside topography and orthoimagery (e.g.
Landesamt für Vermessung und Geoinformation Schleswig-Holstein), the
German authority for climate data DWD or the authority for maritime data
BSH. This data can already be utilized as core for monitoring and
simulating. The INSPIRE-GEOPORTAL[2] and GDI-DE are
considered as useful SDIs on European respectively national level and
MDI-DE as a SDI for maritime data of the German coasts.
Interfaces, data formats and update rates are to be specified to connect
the different hydraulic engineering simulators and the geoportal.
Missing required data (e.g. dike’s soil moisture, water level, swell)
have to be measured by the WGSN. The required spatial and temporal
resolution of the captured data affects the number, the placement, and
the configuration of the sensor nodes.
4.2 Sensor Layer – Geo Sensor Network
A Wireless Geo Sensor Network (WGSN) is implemented for the parameter
sensing on-site. The WGSN consists basically of sensor nodes, which
incorporate geotextiles and environmental monitoring sensors,
communication interfaces as well as the power supply. In this project,
devices with modular design are configured and equipped with different
sensors.
The central aspect is the design of sensor nodes for gathering and
coding the measurements of geotextile sensors and supplementary
environmental sensors. Therefore in the first step, the physical
connection between sensors and the sensor node via standardized
interfaces (I2C, SPI or RS232) and if necessary preadapted electrical
circuits (e.g. geotextile ITA) are prepared. Depending on the required
spatial resolution of the parameters to be sensed, the sensor nodes are
equipped with one or more different sensor units. In a subsequent
calibration phase besides the general test of functionality, the
sensors’ characteristics (resolution, accuracy, characteristic line) are
analyzed. The acquired calibration functions or look-up tables for each
sensor are known and stored in the sensor nodes in order to adjust the
measured data.
After the calibration phase, the sensor nodes are programmed for reading
data series from each single sensor regarding the required temporal
resolution. By means of statistical preprocessing of the collected data
series, an average value and quality measure (e.g. standard deviation)
are encapsulated to data packets. For flexible deployment of the WGSN,
the wireless transmission of the generated packets towards a network
gateway is suggested. Therefore, the sensor nodes are linked to each
other by a radio communication technology that ensures a self-organized
and scalable wireless network. A suitable communication protocol for
that purpose is ZigBee PRO which is based on the physical layer and
media access control protocol IEEE 802.15.4. ZigBee PRO devices can
transmit data over long distances and are able to build a wireless ad
hoc network following a mesh topology. Data packets are forwarded
hop-by-hop according to the routing entries from the intermediate sensor
nodes which act as router in order to reach the network gateway. The
network gateway also acts as a data sink collecting the packets of all
nodes and afterwards transmitting the gathered information to the
integration layer in our architecture.
Based on the ZigBee PRO protocol we use the lately quite popular
Internet of Things (IoT) protocol Message and Query Telemetry Transport
for sensor networks (MQTT-SN)[3] which is
especially useful for Machine-to-Machine communication. An MQTT-SN
message (like a normal MQTT message) consists of a topic and a payload,
so that e.g. a sensor node can publish a message with the topic “soil
temperature” and the payload that consists of the measured temperature
value “4.9 °C”. The advantage of applying this protocol is that each
sensor node can also receive messages from the network.
More precisely, we are using an own extension of the MQTT protocol, the
so-called GeoMQTT protocol. This extension introduces some additional
features compared to the original protocol and is fully compliant with
existing MQTT software. Briefly, a GeoMQTT client adds a timestamp and a
geographic position to every message. The final protocol stack of our
sensor nodes is shown in Figure 4.
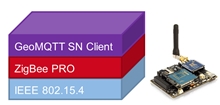
Fig. 4: Protocol stack of one sensor node
Further important aspects to be treated carefully are the
synchronization and the power management of the nodes. Synchronization
is accomplished by a periodical calibration with a master clock from the
network gateway. To achieve a best possible self-sufficient power supply
of the battery-operated nodes, some further aspects have to be taken
into account:
- Adjustment of the
transmission power in the WSN depending on the radio range between the
nodes and channel conditions.
- Application of power
safe control mechanisms which allow the nodes to go from active mode to
sleep mode after transmission. Due to the expected low sensing rates (<
1 sample/min) the nodes can sleep most of the time resulting in long
battery life.
- Utilization of solar
panels for recharging the batteries in case of outdoor application.
4.3 Integration Layer
The integration layer in our proposed architecture merges all the data
from different sources. Therefore we adapt the idea of a sensor bus
(Broering et al. 2010) whereby all the measured data is temporarily
collected in a central component. This bus is basically the second
component of the MQTT protocol which is the so-called message broker.
The broker receives messages from the clients with a specific topic and
disseminates the messages to all clients which are subscribed to the
corresponding topics. This way, applications can simply hook in the bus
and receive all new messages published on a specific topic. The sensor
nodes described in the previous section are directly connected to the
broker and are also able to publish and receive messages. Like mentioned
before, we are using an extension of the original protocol and
therefore, we have implemented a GeoMQTT Broker. This architectural
component is shown in Fig. 5.
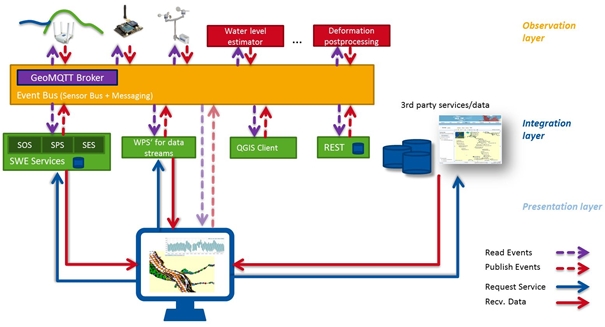
Fig. 5: Event bus as an intermediary layer
However, we try to push the idea of sensor bus even further into a
so-called event bus. This newly introduced concept serve not only as a
bus for sensor data but also for other data like processed sensor data.
Processes can directly hook into the bus waiting for sensor data to
arrive, process this data and republish the results to the same bus.
4.4 Database and Sensor Observation Service
For accessing the captured data the OGC’s SWE standards will be
deployed. For interoperable data management, the OGC standard Sensor
Observation Service (SOS)[4] is used. SOS itself
requires SensorML and O&M standards[5]. The Sensor
Model Language (SensorML)[6] is used to describe
the sensor itself whilst the ISO/OGC Observations and Measurements (O&M)[7]
is aimed for data modelling and encoding. The standard SOS is also part
of a proposal for the INSPIRE maintenance and implementation process for
download services[8]. The data captured by the
installed WGSN is stored in a spatial (geo) database. A feeder
application hooks into the previously described sensor bus and inserts
the arriving data tuples into the database.
For the EarlyDike-project the SOS open source implementation of 52°North
GmbH[9] is to be used. The underlying Geospatial
DBMS PostGIS is a spatial database extension for PostgreSQL
object-relational database. It adds support for geographic objects
allowing location queries to be run in SQL[10].
All used applications are free software according to the GNU General
Public License (GNU GPL)[11].
4.5 Presentation Layer – GeoPortal
The presentation layer is realized as central web-based information
access point called “Dike GeoPortal”. In general, the portal serves for
the presentation of data and invocation of geoprocesses using WMS, WFS
and Web Processing Services (WPS) (Fig. 6). Therefore, the free
mapserver “Geoserver” is deployed providing WMS and WFS of own data
stored in the geo database mentioned above. The geoportal is set up
using the also free software libraries (e.g. OpenLayers[12]
or GeoExt[13]). Base maps (topography, orthoimages
on the landside, bathymetry on the seaside) will be received using WMS
from data providers like the national authorities of the federals states
for spatial base information respectively hydrological data. Further
data, for example sea level and climate/weather will be collected by WFS
from the responsible state institution like BSH respectively DWD. If
necessary or more practical, we will also use SDIs like MDI-DE. Semantic
information is presented in spatially referenced thematic maps overlayed
by dynamic icons on top of the selected base map, Data windows will pop
up if the icons are selected. Time-periods like water levels and gauges
or temperature are visualized by dynamic tables and/or graphs. Data,
measured by the installed WGSN and stored in the mentioned SOS-database,
are accessed for presentation in the geoportal by the SOS requests like
“GetObservation” or “GetResult”. Thus the pure sensor data can be
queried or specific requests e.g. for the last observed value or a graph
of the values of a time-period can be performed. Simulation results are
accessible via WMS, WFS or as a result of WPS.
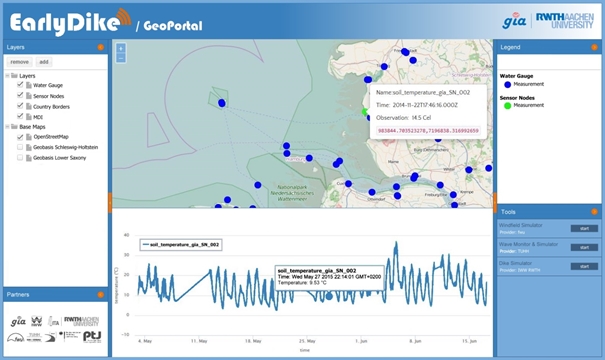
Fig. 6: EarlyDike GeoPortal
For maximizing information efficiency, all connected data (own sensor
data, external data and simulation results) are presented together in
one coherent and spatially referenced matter. Thus, the web portal
delivers descriptions (metadata), provides access, and enables the
map-based visualization of all spatially referenced information. Besides
the current situation, the state in the past can be observed by e.g.
time series to analyze even sudden changes over time.
In addition, we will utilize adaptive software components (like
OpenLayer mobile) to render the dike portal not only for desktop browser
but also for mobile phones (e.g. smartphones). By developing mobile web
forms, users are able to obtain an overview of all sensor values at a
glance, e.g. in case of incoming alert notification. It is also
conceivable to create profiles for addressing different user groups,
e.g. granting access to selected content for public users or providing
access to all data for expert users.
5. SUMMARY AND OUTLOOK
The development of an SSDI is an essential part of an early warning
system for floods and storm surges and enables the integration of all
relevant data in a fully digital workflow including data capturing
on-site, embedding external data sources as well as the inclusion of
hydro engineering simulation tools. This leads to a completely novel
approach for a holistic dike monitoring as a general basis for early
warning systems. The outcome of the work packages will increase
knowledge in geo information science since it directly affects future
developments in geospatial standardization towards spatial modeling and
spatial data integration, especially concerning water and hydraulic
engineering.
The complete warning system will protect against uncontrolled flooding
of wide areas. In the worst case of unavoidable dike failure, it will
give early alert and inundation prediction. It will be implemented first
on a test dike at the German coast of the North Sea. In case of a
successful evaluation designated users are the German authorities for
coastal protection in Schleswig-Holstein (LKN Husum) and in Lower Saxony
(NLWKN Norden). They are corresponding partners in this project. Other
dikes around the whole world may benefit from such an early warning
system for saving lives and economic values.
ACKNOWLEDGEMENTS
The EarlyDike project (http://www.earlydike.de/) is funded by the German
Federal Ministry of Education and Research (BMBF) within the
GEOTECHNOLOGIEN programme (03G0847A).
REFERENCES
Akyildiz, I.F.; Vuran, M.C. (2010): Wireless Sensor Networks. John
Wiley, West Sussex, UK,
Benedict, K. (2005): The Open Geospatial Consortium Web Map, Web Feature
and Web Coverage Service Standards – an Overview. ESIP Federation
Meeting, Mexico.
Bernard, L.; Kanellopoulos, I.; Annoni, A. (2005): The European
geoportal – one step towards the establishment of a European Spatial
Data Infrastructure. Computers, environment and urban systems, 29(1).
Bocher, E., Neteler, M. (2012): Geospatial Free and Open Source Software
in the 21st Century, Dordrecht.
Broering, A., Foerster, T., Jirka, S., Priess, C. (2010): Sensor Bus: An
Intermediary Layer for Linking Geosensors and the Sensor Web. In:
Proceedings of the 1st International Conference on Computing for
Geospatial Research and Application, COM.Geo’10, 21-23 June, Bethesda,
MD, USA; ACM: New York. pp. 1-8.
Craglia, M.; Annoni, A. (2006): INSPIRE: an innovative approach to the
development of spatial data infrastruc-tures in Europe. In: Proc. of
GSDI World Conference, Santiago, Chile.
BKG (2013): Federal Agency for Cartography and Geodesy: Ad hoc reporting
by INSPIRE AG to the GDI-DE Steering Committee (LG GDI-DE): INSPIRE
Infrastructure for Spatial Information in Europe. Member State Report:
Germany.
Groot, R. (2003): Geospatial Data Infrastructure: Concepts, Cases, and
Good Practice, Oxford, UK,
Grothe, M., Kooijman, J. (eds.) (2008): Sensor Web Enablement.
Netherlands Geodetic Commission, Netherlands, 2008
Harvey, F.; Iwaniak, A.; Coetzee, S; Cooper A.K. (2012): SDI Past,
Present and Future: A Review and Status Assessment. In: Proc. of GSDI 13
World Conference, Quebec, Canada,
INSPIRE (2007): Directive 2007/2/EC of the European Parliament and of
the Council of 14 March 2007 establishing an Infrastructure for Spatial
Information in the European Community (INSPIRE),
http://eur-lex.europa.eu/LexUriServ/LexUriServ.do?uri=CELEX:32007L0002:EN:NOT.
Iyengar, S. S.; Brooks, R. R. (2012): Distributed Sensor Networks. CRC
Press: Boca Raton, USA.
Jirka, A; Bröring, A.; Stasch, C. (2009): Applying OGC Sensor Web
Enablement to Risk Monitoring and Disaster Management. In: Proc. of GSDI
11 World Conference, Rotterdam, Netherlands.
Lehfeldt, R., Melles, J. (2011): Die Marine Dateninfrastruktur
Deutschland MDI-DE. In: Karl-Peter Traub, Jörn Kohlus und
Thomas
Lüllwitz (Hg.): Geoinformationen für die Küstenzone. Norden Halmstad:
Points Verlag (3), S. 107–116.
Pengel, B. et al. (2013): Flood Early Warning System: Sensors and
Internet. In: IAHS Red Book N 357, Floods: From Risk to Opportunity.
IAHS Publ. 357.
Rüh, C; Bill, R. (2012): Concepts, Models and Implementation of the
Marine Spatial Data Infrastructure in Germany (MDI-DE). In: Proc. of
ISPRS Congress, Sydney, Australia, 2012
Schleyer, A.; Luckhardt, T.; Thiel, F.,Welzel, R.-W. (2014):
Geodateninfrastruktur. In: Kummer et al. (2014): Das Deutsche
Vermessungs- und Geoinformationswesen – Band 2015. Wichmann Verlag,
Heidelberg, S. 829-871, ISBN 978-3-87907-547-8
Schüttrumpf, H. (2008): Aktuelle Entwicklungen im internationalen
Küsteningenieurwesen - International Conference on Coastal Engineering.
In: Korrespondenz Wasserwirtschaft : KW. - Hennef : GFA. - ISSN:
1616-430X, 1865-9926. - 1 (2008) 12, S./Art.: 664-665.
Spies, K.-H.; Heier, C. (2010): Sensor Web Enablement in der
Wasserwirtschaft – Von der Hydrologie über das Monitoring zum
Management. In: Schilcher (Hg.): Geoinformationssysteme: Beiträge zum
15. Münchner Fortbildungsseminar. abcverlag, Heidelberg,
Thomas, R. (2013): European Directive (2007/2/EC) INSPIRE - Update on
INSPIRE Directive. UN Expert Group on Resource Classification, Fourth
Session, 23- 26 April 2013.
https://www.unece.org/fileadmin/DAM/energy/se/pp/unfc_egrc/egrc4_april2013/25_april/
5_Thomas_INSPIRE.pdf
Walter, K., Nash, E. (2009): Coupling Wireless Sensor Networks and the
Sensor Observation Service – Bridging the Interoperability Gap. In:
Proc. of 12th AGILE International Conference on Geographic Information
Science, Hannover, Germany.
CONTACTS
Univ.-Prof. Dr.-Ing. Jörg Blankenbach
Dr.-Ing. Ralf Becker
M.Sc. Stefan Herle
RWTH Aachen University
Geodetic Institute and
Chair for Computing in Civil Engineering & Geo Information Systems
Mies-van-der-Rohe-Str. 1
D-52074 Aachen
GERMANY
Tel. + 49-241-80-95300
Fax + 49-241-80-92142
Email: {blankenbach | ralf.becker | herle}@gia.rwth-aachen.de
Website: http://www.gia.rwth-aachen.de
Univ.-Prof. Dr.-Holger Schüttrumpf
RWTH Aachen University
Institute of Hydraulic Engineering and Water Resources Management
Mies-van-der-Rohe-Str. 17
D-52056 Aachen
GERMANY
Tel. + 49-241-80-25262
Fax + 49-241-80-22348
Email: schuettrumpf@iww.rwth-aachen.de
Website: http://www.iww.rwth-aachen.de
Dipl.-Ing. Till Quadflieg
RWTH Aachen University
Institut fuer Textiltechnik
Otto-Blumenthal-Str. 1
D-52074 Aachen
GERMANY
Tel. + 49-241-80-23400
Fax + 49-241-80-22422
Email: till.quadflieg@ita.rwth-aachen.de
Website: http://www.ita.rwth-aachen.de
Dr.-Ing. Rainer Lehfeldt
Federal Waterways Engineering and Research Institute
Wedeler Landstr. 157
D-22559 Hamburg
GERMANY
Tel. +49-40-81908312
Fax.: +49-40-81908373
Email: rainer.lehfeldt@baw.de
Website: http://www.baw.de
Univ.-Prof. Dr.-Ing. Jürgen Jensen
University of Siegen
Research Institute for Water and Environment
Paul-Bonatz-Str. 9-11
D-57076 Siegen
GERMANY
Tel. + 49-271-7402172
Fax. +49-271-7402722
Email: juergen.jensen@uni-siegen.de
Website:
http://www.uni-siegen.de/fb10/fwu/wb/
Univ.-Prof. Dr.-Ing. Peter Fröhle
Hamburg University of Technology
Insitute of River and Coastal Engineering
Denickestr. 22
D-21073 Hamburg
GERMANY
Tel. +49-40-428783463
Fax. +49-40-428782802
Email: froehle@tuhh.de
Website: http://www.tuhh.de
[1]
http://www.opengeospatial.org/ogc
[2]
http://inspire-geoportal.ec.europa.eu/
[3] www.mqtt.org
[4]
http://www.opengeospatial.org/standards/sos
[5]
http://inspire.ec.europa.eu/events/conferences/inspire_2014/pdfs/18.06_5_16.00_Simon_
Jirka.pdf
[6]
http://www.opengeospatial.org/standards/sensorml
[7]
http://www.opengeospatial.org/standards/om
[8]
Sos_In_The_Conte xt_Of_Inspire.pdf>
[9]
http://52north.org/communities/sensorweb/sos/
[10]
http://postgis.net/
[11]
http://www.gnu.org/licenses/licenses.en.html
[12]
http://openlayers.org/
[13]
http://geoext.org/